Properties of Laterized Concrete Incorporating Sawdust Ash as A Partial Replacement for Cement
Author(s): ODUBELA Christiana Adebola and Oluwatobi Gbenga Aluko*
Abstract
Ordinary Portland cement (OPC), the main binder in concrete, is the most expensive component and the major contributor to the embodied carbon of the composite. This necessitates alternative eco-friendly materials such as waste, with comparative binding properties as a part replacement for OPC to have economic and sustainable concrete production with minimal hazardous impact on the environment. This study uses Sawdust Ash (SDA) which is a waste product of the wood milling industry, to replace some proportion of OPC in concrete. To further reduce the cost and negative environmental impact of concrete, laterite was used to replace river sand, and fresh and hardened properties were investigated. The fresh property evaluated in this study is the slump, while the hardened properties investigated are the density and compressive strength. This study showed the viability of successfully incorporating SDA as a partial replacement of OPC and laterite soil to replace the conventional fine aggregate. An acceptable slump and compressive strength can be achieved using 10% SDA and 45% laterite as partial replacement of OPC and river sand, respectively.
Introduction
The production of concrete in large quantities for different
applications worldwide is a major sustainability concern [1].
This detrimental effect on the environment results from the high
emission produced during the production of the major binder (i.e.,
ordinary Portland cement) in concrete. An equivalent amount of
carbon dioxide is emitted into the environment just o produces 1
tonne of ordinary Portland cement (OPC). In addition, OPC is the
most expensive and energy-intensive component in concrete. As
more rapid development will ensue in the coming years in rural
areas and developing countries, more production of OPC and
corresponding emission is anticipated. Therefore, to ensure that
future constructions in these areas are economical, resilient, and
sustainable, it is paramount to find alternative locally available
materials that can be used to replace OPC and other materials in
concrete.
Several studies have explored using different materials as
partial replacement of OPC. However, most of these materials
are industrial by-products such as slag, silica fume, fly ash, rice
husk ash [2-4]. However, some of these materials are not locally
available in rural areas of developing countries. For constructions
in these areas to be sustainable conscious, alternative readily
available waste materials from the agricultural industry can be
utilized [5-8]. This study explored possible locally available
materials that can be used to produce eco-friendly concrete in
rural areas of Nigeria. One of the materials that can replace OPC,
readily available in these areas, is sawdust ash (SDA). SDA is
obtained by burning at a specific high temperature the sawdust,
which is a solid waste product of timber milling. The use of SDA
as a partial replacement for OPC not only proffers an alternative
binder but also helps to manage the detrimental waste that is a
major environmental threat in these areas and as well mitigate
greenhouse emissions caused by the production of cement [8].
The emergence of Laterized concrete can be traced back to
the 1970s, when the pioneering researcher, published many
researches works on laterized concrete and currently still gaining
expanding interest from many researchers because of its strength
characteristics and sustainable prospects [9-13]. Some studies
have explored the use of SDA as a partial replacement of OPC
in concrete, and even some studies utilized SDA and laterite
as aggregate in concrete [8,14-17]. However, to the best of the
authors? knowledge, there is a limited to no study on using SDA
to replace OPC in laterized concrete partially. Therefore, the study
explored the major engineering properties of laterized concrete
incorporating SDA alongside determining the optimum mix for
the components. SDA normal-weight as a partial replacement
of OPC, and laterite is used to replace the conventional fine
aggregate partially. This research is innovative as it investigates
the viability of using locally available materials as components in
concrete. It is anticipated that this study will open more pathways
for research and the application of sustainable building materials.
It is also hoped that this research will propel more economical and
sustainable development in rural areas and developing countries.
Methodology
Type 1 OPC was used as the main binder, and it complies with ASTM C150. The chemical compound of the cement is shown in
Table 1 as obtained from the manufacturer. SDA was used as a partial replacement of OPC in the range of 0 to 15% at increments of
5%. The OPC is obtained from a local building supplier, and the sawdust was obtained from Itamaga and Ijebu ode sawmill plants.
After retrieving the sawdust from the local sawmill plant, it was burnt in the open air for 48 hours to obtain the ash (i.e., SDA). The
SDA was sieved to obtain particles passing through the 75μm sieve, and the observed color of the SDA was greyish.
Granite was used as the only coarse aggregate in all mixtures while laterite is used as a partial replacement of river sand as fine
aggregate in the range of 0 to 45 % at increments of 15%. All aggregates used in this study are obtained from the ground?s laterites
and fine aggregate from Ikorodu and coarse aggregate from quarry in Ijebu ode. The sieve analysis of the aggregates used in this
study is presented in Figure 1, and their specific gravity and bulk density are presented in Table 2. It will be observed from Figure 1
that the laterite soil is finer than the conventional fine aggregate, but the gradation is similar. The water used for this study is potable,
and it conforms with for concrete mixtures [18].
Table 1: Chemical Composition of Opc
Constituent |
Weight (%) |
Lime (CaO) |
64.64 |
Silica (SiO 2
) |
21.28 |
Alumina (Al 2
O 3
) |
5.60 |
Iron Oxide (Fe2
2O3
) |
3.36 |
Magnesia (MgO) |
2.06 |
Sulphur Trioxide (SO3
) |
2.14 |
Total Alkalis |
0.05 |
Insoluble Residue |
0.22 |
Loss of Ignition |
0.92 |
Lime Sarturated Factor |
0.92 |
Silica Modulus |
2.38 |
Table 2: Specific Gravity and Bulk Density of Materials
Material |
OPC |
River sand |
River sand |
Granite |
Specific gravity |
3.05 |
2.40 |
2.40 |
2.85 |
Bulk density (Kg/m 3
) |
1440 |
1437 |
1436 |
1437 |
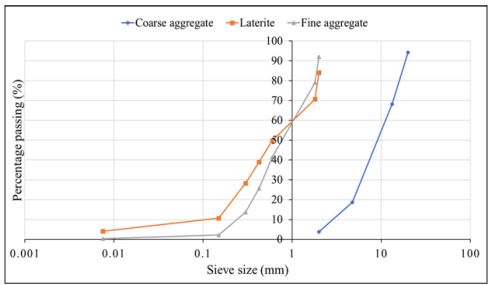
Figure 1: Sieve Analysis of Aggregates
The mixtures proportions evaluated in this study are classified in terms of percentage and presented in Table 2. The w/cm indicates
the water to the cementitious ratio where the cementitious component is composed of the mass of all the binder used (i.e., OPC and/
or SDA)
Table 2: Mixture Proportions (%)
Mixture notation |
Mixture ID |
Binder |
Fine aggregate |
w/cm |
OPC |
SDA |
River sand |
Laterite |
M1 |
100OPC |
100 |
- |
100 |
- |
0.63 |
M2 |
100OPC15LTR |
100 |
- |
85 |
15 |
0.63 |
M3 |
100OPC30LTR |
100 |
- |
70 |
30 |
0.63 |
M4 |
100OPC45LTR |
100 |
- |
55 |
45 |
0.63 |
M5 |
5SDA15LTR |
95 |
5 |
85 |
15 |
0.63 |
M6 |
5SDA30LTR |
95 |
5 |
70 |
30 |
0.63 |
M7 |
5SDA45LTR |
95 |
5 |
55 |
45 |
0.63 |
M8 |
10SDA15LTR |
90 |
10 |
85 |
15 |
0.63 |
M9 |
10SDA30LTR |
90 |
10 |
70 |
30 |
0.63 |
M10 |
10SDA45LTR |
90 |
10 |
55 |
45 |
0.63 |
M11 |
15SDA15LTR |
85 |
15 |
85 |
15 |
0.63 |
M12 |
15SDA30LTR |
85 |
15 |
70 |
30 |
0.63 |
M13 |
15SDA45LTR |
85 |
15 |
55 |
45 |
0.63 |
Like the conventional mixing method, the aggregates and binders were first dry-mixed by a mixing machine (Figure 2a) for 3 mins,
followed by the addition of water gradually to the mix. The mixture was further mixed for 2 mins after the addition of water. After
mixing, the slump test was carried out following (Figure 2b), and the pre-prepared mold was filled with the concrete mixture (Figure
2c) [19]. All specimens are prepared and tested according to several British standards. The compressive test was carried out following
[20]. Four 150 x 150 x 150mm cubes were cast for each mixture for the compressive strength testing (Figure 2d). The slump test was
measured immediately after mixing. All samples are cured underwater after de-molding until the required tests are due (i.e., 7, 14,
and 28 days). The density and compressive tests were carried out at 7, 14 and 28 days after curing.
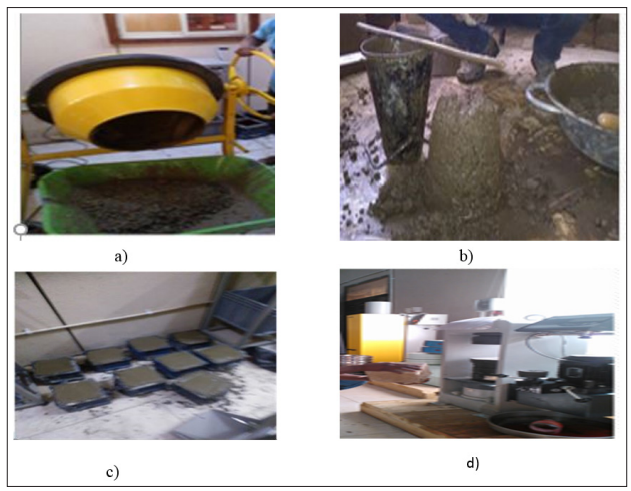
Figure 2: Experimental Method a) Machine Mixing of Concrete b) Slump Test c) Casting of Cubes For Compressive Strength d)
Crushing Testing
Results and Discussion
Fresh Properties
Slump
The slump of concrete is one of its main fresh properties. The slump of concrete indicates its consistency and how workable the
mixture is. The effect of partial replacement of OPC with SDA and river sand with laterite is presented in Figure 3 a-d. It will be
observed that in all proportions of OPC, increasing the amount of laterite sand reduces the slump of the mixture. The reduction in the slump with the incorporation of laterite soil might result from its higher absorption rate, which reduces the amount of free water
available in the mixture. When 100% OPC is used, the slump is reduced by approximately 50% when the river sand is replaced with
45% laterite soil. A similar trend was observed when OPC was replaced with SDA for 15%.
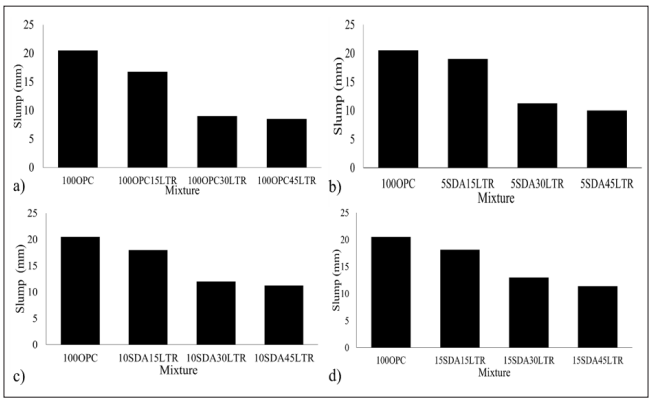
Figure 3: Effect of Replacement of River Sand at Different Levels When Opc Used Was a) 100% OPC b) 95% c) 90% d) 85%
Hardened Properties
Density
The results presented in Figure 4 show the hardened density of the mixtures measured at 7, 14 and 28 days. It will be observed from
the figure that all mixtures have approximately the same density of about 2400Kg/m3
except for M11 and M12. As all the densities
measured are within the range of 2400Kg/m3
, this showed that all the concrete mixtures considered in this study could be classified
as normal-weight concrete.

Figure 4: Effect of Sda and Laterite on The Density of Concret
Compressive strength
The compressive strength of concrete indicates its ability to withstand a load in compression and can be correlated to other mechanical
properties of concrete. For this experimental study, 52 cubes samples with a dimension of 150mm were tested for compressive strength.
The compressive strength results presented in Figure 5 is an average of 4 cube samples for each mixture. It will be observed for all
mixtures that the compressive strength increases with age. This increasing compressive strength shows the progression of hydration
reaction with time. For all mixtures incorporating only OPC as a binder (i.e., M1 to M4), it will be observed that the compressive
strength at seven days is about 65% of that at 28 days and 14 days? strength is about 83% of the 28 days compressive strength. The
incorporation of SDA as partial replacement of OPC resulted in a significant decrease in the compressive strength of the concrete
samples at all ages. This observation can result from a possible low pozzolanic reaction of the SDA. However, increasing the content
of SDA from 5% to 10% resulted in compressive strength similar to that of the control without SDA. This observation is similar to
other studies in which silica fume and metakaolin are used to replace OPC and the optimum was found to be 10% [21- 22]. In contrast
to the effect of partial replacement of OPC with SDA, it will be observed from Figure 4 that increasing the replacement level of river
sand with laterite sand increases the compressive strength significantly. Based on these results, the optimum replacement of OPC
and river sand respectively is 10% SDA and 45% laterite sand (i.e., M10) [23-25].
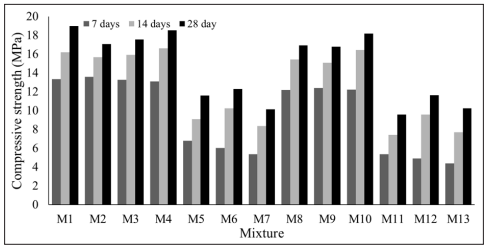
Figure 5: Effect or Sda And Laterite on The Compressive Strength of Concrete
Conclusion
This study explored the major properties of concrete incorporating
SDA and laterite as partial replacement of OPC and fine aggregates,
respectively. Based on this experimental study, the following
conclusion could be drawn;
- Replacement of OPC with SDA in the range of 5 to 15% and
river sand with laterite sand in the range of 15 to 45% have
no significant impact on the workability of concrete mixtures.
- Laterized concrete incorporating SDA as a partial replacement
of OPC can be classified as a normal weight concrete as all
the densities measured are about 2400Kg/m3
.
- The optimum amount of SDA is 10%, and that of laterite is
45% in terms of compressive strength
- More research should be explored in this area to observe how
incorporating different amounts of SDA and laterite affects
other mechanical and durability properties of concrete.
References
- Aluko O, Awolusi T, Adesina A (2020) Influence of Curing
Media and Mixing Solution on the Compressive Strength of
Laterized Concrete. Silicon 12: 2425-2432.
- Adesina A (2018) Use of rice husk ash in concrete: a review
of mechanical properties. J Curr Constr Issues 3: 15-22.
- Adesina A, Awoyera (2019) Overview of trends in the
application of waste materials in self-compacting concrete
production. P. SN Appl. Sci 1: 962.
- Rupali SP, Rakesh KP, Bibhuti B M, Tanish D (2018)
Influence of incorporation of rice husk ash and coarse recycled
concrete aggregates on properties of concrete. Construction
and Building Materials 173: 289-297.
- Sojobi AO, Aladegboye OJ, Awolusi TF (2018) Green
interlocking paving units. Construction and Building
Materials 173: 600-614.
- Sojobi AO (2016) Performance of eco-friendly lightweight
interlocking concrete paving units incorporating sawdust
wastes and laterite. Cogent Engineering 3: 1-27.
- Sivakrishna A, Adesina A, PO Awoyera, K Rajesh Kumar
(2019) Green concrete: A review of recent developments,
Materials Today: Proceedings 27: 54-58.
- ER Teixeiraa, A Camõesa, FG Brancob. (2019) Valorisation
of wood fly ash on concrete. Resources, Conservation &
Recycling 145: 292-310.
- Balogun L A, Adepegba D (1982) Effect of varying sand
content in laterized concrete. International Journal of Cement
and Composite and Lightweight Concrete 4: 235-240.
- Udoeyo F F, Iron U H, Odim O O (2006) Strength performance
of laterized concrete. Construction and Building Materials.
20: 1057-1062.
- Salau MA (2003) Long-term deformations of laterized
concrete short columns. Building and Environment 38: 469-
477.
- Awoyera PO, Akinmusuru JO, Dawson AR, Ndambuki JM,
Thom NH (2018) Microstructural characteristics, porosity and
strength development in ceramic-laterized concrete. Cement
and Concrete Composites 86: 224-237.
- Chowdhury S, Maniar A, Suganya OM (2015) Strength
development in concrete with wood ash blended cement
and the use of soft computing models to predict strength
parameters. Journal of Advanced Research 6: 907-913.
- Awolusi T F, Sojobi A O, Afolayan J O (2017) SDA and
laterite applications in concrete: Prospects and effects of
elevated temperature. Cogent Engineering 4: 1.
- Subhash C Yaragal, SN Basavana Gowda, C Rajasekaran
(2019) Characterization and performance of processed
lateritic fine aggregates in cement mortars and concretes,
Construction and Building Materials 200: 10-25.
- Olumoyewa A D, Aladegboye Oluwasegun J, Odeyemi
Sheyanu V (2018) Evaluation of Laterized Earth Moist
Concrete in Construction Works, International Journal of
Civil Engineering and Technology 9: 327-333.
- Olumoyewa A, Odeyemi Samson O, Orama Joy A (2018)
Experimental data on the splitting tensile strength of bamboo
reinforced lateritic concrete using different culm sizes, Data
in Brief 20: 1960-1964
- BS 3148 (1980) Methods of Test for Water for Making
Concrete. British Standard Institution, London.
- BS EN 12350-2 (2009) Testing Fresh Concrete. Slump-Test.
British Standard Institution, London.
- BS EN 206 (2013) Concrete - Specification, performance,
production and conformity, British Standards Institution,
London.
- Akcay B, Tasdemir M (2018) Performance evaluation of
silica fume and metakaolin with identical finenesses in selfcompacting and fibre reinforced concretes. Construction and
Building Materials 185: 436-444.
- Yazici H (2008) The effect of silica fume and high-volume
Class C fly ash on mechanical properties, chloride penetration
and freeze-thaw resistance of self-compacting concrete.
Construction and Building Materials 22: 456-462.
- Elinwa A, Ejeh S, Mamuda A (2008) Assessing of the fresh
concrete properties of self-compacting concrete containing
sawdust ash. Construction and Building Materials 22: 1178-
1182
- Ukpata J O, Maurice E E, Akeke G A (2012) Compressive
Strength of Concrete Using Lateritic Sand and Quarry Dust
as Fine Aggregate, ARPN Journal of Engineering and Applied Sciences 7: 1.
- Salem M E (2009) Performance of Laterite Aggregate
Concrete. M.Eng Thesis, Dept. of Civil Engineering,
Universiti Teknologi, Malaysia.
View PDF